Over the last decade, there has been a surge in demand for high-speed data communication, with exponentially increasing data rates. The advent of 5G wireless communication technology enables faster data transfer, higher bandwidth, lower latency and greater reliability than its predecessors. This, in turn, will support various emerging applications, including autonomous vehicles, smart-city infrastructure and augmented reality. However, implementing this new technology efficiently requires advanced electronic components and materials that can operate at high frequencies and power levels with minimal energy consumption. One of those technologies is wide-bandgap (WBG) semiconductors.
Semiconductor materials play a vital role in the performance of electronic components for wireless communication. Traditional semiconductors, such as silicon (Si), gallium arsenide (GaAs) and indium phosphide (InP), are limited in handling high-voltage, high-frequency and high-temperature operation. Therefore, there is a growing interest in exploring and adopting new materials with superior electrical and thermal properties, such as WBG semiconductors. WBG devices can enable higher efficiency, power density and operation at higher frequencies than traditional semiconductors.
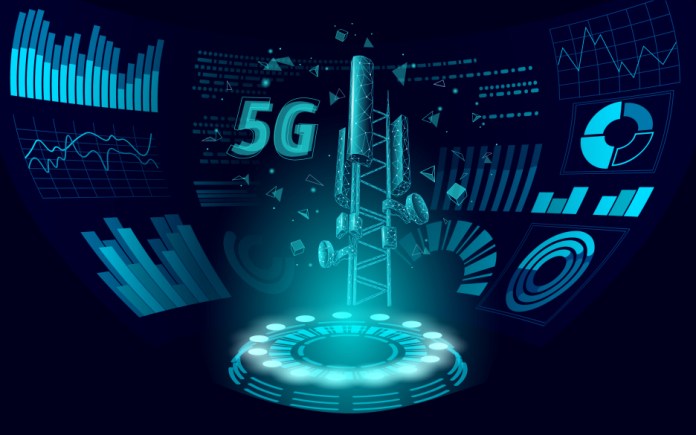
5G wireless will support emerging applications, including autonomous vehicles, smart cities and augmented reality. (Source: Shutterstock)
Benefits of WBG in 5G applications
WBG semiconductors have unique properties that make them suited for 5G applications. One key advantage of WBG semiconductors, such as silicon carbide (SiC) and gallium nitride (GaN), is their ability to handle high voltages and operate at high frequencies.
In 5G networks, high-frequency signals transmit large amounts of data at high speeds. WBG semiconductors can operate at these high frequencies without excessive power loss or signal distortion. This allows for more efficient and reliable communication between devices in the network.
Another advantage is their ability to operate at high temperatures. In 5G applications, high temperatures can be generated due to the high power levels required for signal transmission. WBG semiconductors can tolerate more heat and radiation without suffering damage, increasing their reliability in high-temperature environments.
In addition, WBG semiconductors have faster switching speeds than traditional Si-based semiconductors. This means they can switch on and off more quickly, allowing faster data transfer and greater energy efficiency.
RF GaN properties
The key physical properties that make GaN an excellent semiconductor for 5G applications include the following:
- High electron mobility translates into higher switching frequencies and faster switching rates that can be achieved more easily without damaging the device.
- High breakdown voltage allows for higher power density and smaller device sizes (the die active area is reduced compared with other semiconductors).
- Low on-resistance (RDS(on)), as low as a few milliohms, depending on the specific device, results in less power loss and improved efficiency.
- Excellent thermal conductivity means GaN-based RF devices like amplifiers and transmitters (especially in the gigahertz band) can tolerate elevated operating temperatures without performance degradation. Additionally, the need for added cooling components can be reduced, reducing the weight and size of the solution.
RF SiC properties
SiC is another WBG semiconductor suitable for 5G applications due to its unique physical properties, such as high thermal conductivity, wide bandgap and high breakdown voltage. SiC offers higher electron mobility and saturation velocity than silicon, allowing for faster switching speeds, higher power density and better efficiency.
SiC also has a significantly higher operating temperature than silicon, allowing it to operate at higher temperatures without performance degradation, which is crucial for high-power RF electronics. SiC has better thermal conductivity than GaN, making it a suitable material for use in high-temperature RF power applications.
WBG trends in RF
In RF, specifically for 5G applications, WBG semiconductors are becoming increasingly prevalent due to their ability to handle high-frequency signals and high power levels. Here are some major trends in WBG semiconductors for 5G applications.
Power amplifiers
Power amplifiers (PAs) are a critical component in 5G networks. WBG semiconductors like GaN are becoming increasingly common because they can operate at higher frequencies and handle higher power levels than traditional Si-based PAs. Most of this market is dominated by GaN-on-SiC technology, which can compete with more established technologies like LDMOS and GaAs, benefitting from the transition to 6-inch wafer production.
Microchip Technology Inc. has adopted GaN-on-SiC technology in its ICP2637 PA. Operating in the 23- to 30-GHz frequency range, this device is a three-stage MMIC PA capable of delivering 37-dBm output power and 25-dB small signal gain. With a typical power-added efficiency of 40% and a maximum channel temperature of 275°C, the ICP2637 is suitable for 5G, satellite communications and aerospace and defense applications.
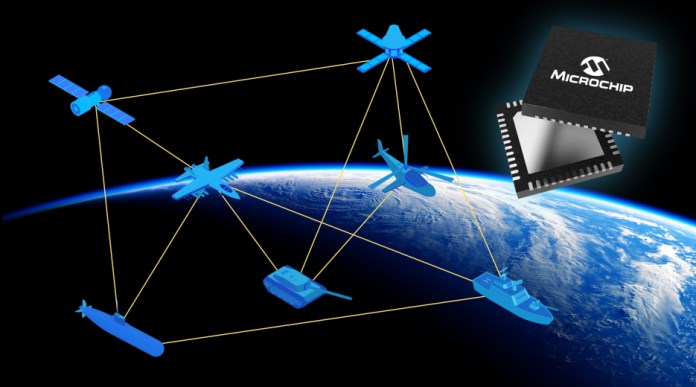
Microchip’s GaN RF power products are fabricated using GaN-on-SiC technology for a combination of high power density and high-voltage operation. (Source: Microchip Technology)
Another example is Qorvo’s QPA2211, a Ka-band PA built on GaN-on-SiC 0.15-µm process technology. Suitable for 5G networks and satellite communications, this PA operates on the 27.5- to 31-GHz frequency band, achieving 5-W linear power. The package’s base is pure copper to offer improved thermal management.
Base-station power supplies
Base stations require high-efficiency power supplies in wireless infrastructure to minimize power consumption and reduce operating costs. Traditionally, RF PAs employ LDMOS transistors to produce kilowatts up to a few gigahertz, making them inefficient. GaN devices for lower-power/higher-volume small-cell installations are employed to improve efficiency at 5G frequencies. LDMOS employs 26- to 32-V DC rails and GaN uses 50–60 V. RF PAs are only 60% efficient. Therefore, every watt saved producing power rails from a battery-backed system supply, such as 48 V, is important.
Envelope tracking is increasingly used to match the PA supply voltage to the modulating signal’s amplitude, thus maximizing system efficiency. Due to the high modulation frequencies (tens of megahertz), traditional DC/DC converters cannot be quick enough. An external envelope-tracking circuit using GaN transistors for high-speed dynamic tracking may be the right solution to pulse the drain current to the RF amplifier.
SiC power discretes and modules greatly enhance the performance of power supplies in base stations. Microchip’s MSCSM120VR1M062CT6AG-Module, for instance, is a SiC MOSFET Vienna rectifier power module featuring an extremely low RDS(on) of just 5 mΩ. This module is a high-efficiency converter operating at high operating frequencies and temperatures.
In addition, the integrated SiC Schottky diode ensures temperature-independent switching behavior. The device, suitable for application in switched-mode power supplies and PFC stages, is rated for 1,200 V/420 A and can be directly mounted to the heat sink. It embeds an internal thermistor for temperature monitoring.
Advanced antenna systems
The use of advanced antenna systems (AASes) is a key factor in achieving the high data rates and low latency required by 5G, and WBG semiconductors are increasingly used in their development. High-performance amplifiers are required for AASes, and GaN-on-SiC is the technology of choice, thanks to its high efficiency in high-power and high-frequency conditions.
AASes are antenna systems that exploit the antenna array technology to improve the performance and spectral efficiency of 5G transceivers. These systems include adaptive beamforming, multiple-input multiple-output (MIMO) and space-division multiple access (SDMA).
Active AAS is the predominant technology in higher-frequency bands, such as near-millimeter–wave (near-mmWave) bands. GaN HEMT PAs are preferred in AAS applications, as they achieve a very high power density, thus meeting the challenging requirements of space, weight and thermal management of 5G high-frequency applications.
Given its tiny size and high RF power output, GaN is suited for the next generation of active electronically steered array (AESA) radars and various other applications. GaN offers big potential benefits in these applications, and power densities of at least 20 W/mm are expected to be attainable in the not-too-distant future.
mmWave band
5G networks operating in the mmWave band require components that handle high frequencies and power levels. WBG semiconductors like GaN-on-SiC are well-suited for this application due to their high operating frequency and power-handling capabilities.
This technology offers some big advantages over silicon, such as the ability to handle higher voltage in a smaller area and cover a wider range of frequencies. It also offers high power density, a key factor for implementing the multiple antenna systems required by 5G. To create a narrow beam, beamforming requires a higher frequency. This results in greater attenuation and the need for many antennas.
Advertisement
Learn more about Microchip TechnologyQorvo