A reliable quantum internet is now one step closer to reality. A research team at Stony Brook University is successfully tackling the major challenges of building a quantum internet testbed by demonstrating a foundational quantum network measurement that uses room-temperature quantum memories.
Quantum networks enable a new level of non-hackable security, even from more powerful quantum computers. These systems will eventually bring quantum information and entanglement across many nodes and long distances. There are hurdles, however, the main one being the development of quantum repeaters, which the researchers call “one of the more complex challenges in current physics research.” Quantum memories play a critical role in these repeaters.
The Stony Brook team recently announced that it built and characterized quantum memories, operating at room temperature. They also demonstrated that these memories have identical performance, which will be key to building large-scale quantum repeater networks that will be made up of several of these memories.
The researchers demonstrated that storage and retrieval of optical qubits in room-temperature quantum memories won’t significantly distort the joint interference process. Instead, it will allow for memory-assisted entanglement swapping to distribute entanglement over long distances—a key to building operational quantum repeaters. Their findings are described in a paper published in the Nature journal “Quantum Information.”
The Stony Brook research team is comprised of Eden Figueroa, a Stony Brook Presidential Innovation Endowed Professor and Director of the Center for Distributed Quantum Processing; Sonali Gera, a post-doctoral researcher; and Chase Wallace, a Ph.D. student. Figueroa, who is the lead author, holds a joint appointment at the U.S. Department of Energy’s Brookhaven National Laboratory.
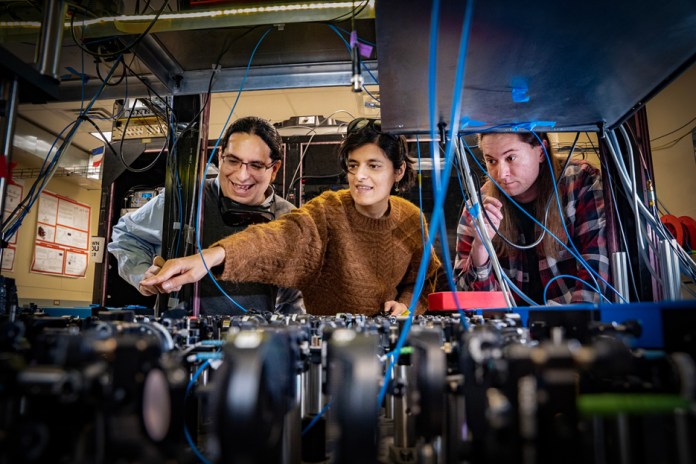
Lead researcher Eden Figueroa (left) with Sonali Gera and Chase Wallace in the Stony Brook Quantum Information Laboratory (Source: John Griffin, Stony Brook University)
The research team also collaborated with scientists from Qunnect Inc., a Brooklyn, New York–based quantum technology spinoff from Stony Brook University, and with international colleagues from the University of Padova in Italy.
The importance of repeaters and room temperature
Quantum repeater networks require independent quantum memories to store and retrieve indistinguishable photons to perform high-repetition entanglement swapping operations.
In Figure 1, a quantum repeater hop requires two sources of entangled photon pairs separated by distance L (infinite symbols). One photon from each pair is sent toward a central measurement node (shaded area), where they are stored in quantum memories. Partner photons are sent in opposite directions, also stored in quantum memories. A measurement quantifying the indistinguishability of the two photons arriving at the central node is used to entangle distantly located photons.
By stitching together several of these repeater hops, it is possible to spread entanglement over hundreds of kilometers, according to the researchers.
The researchers tested how identical these memories are functionally by sending identical quantum states into each of the memories and using the Hong-Ou-Mandel Interference process on memory outputs, a standard test quantifying the indistinguishability of photon properties.
As noted earlier, the team demonstrated that the process of storing and retrieving optical qubits in room-temperature quantum memories does not significantly distort the joint interference process and allows for memory-assisted entanglement swapping to distribute entanglement over long distances. Doing this at room temperature is critical to achieving large-scale quantum networks.
Quantum hardware developed by the team operates at room temperature, which lowers the cost of operation and speeds up the system. Much quantum research today, however, is performed at temperatures near absolute zero, which is more expensive, slower and more challenging to the network, the researchers said.
To date, the team has received U.S. patents for quantum storage at room temperature and high-repetition–rate quantum repeaters. The researchers believe their work is unique.
Remaining hurdles
The team’s next steps are to build and characterize sources of entanglement compatible with quantum memories and design mechanisms to “herald” the presence of stored photons across many quantum memories. There are still several hurdles.
According to Wallace, “The main challenges are the problem of source-memory compatibility. The leading way to produce pairs of entangled photons at high rates is via a process known as down-conversion, which inherently produces a pair of photons that are extremely broadband in frequency. Quantum memories, rather annoyingly, won’t talk to any light that is broadband, as atoms are typically very picky about what frequency of light they interact with.”
Wallace further explained that the team’s approach is to reduce the linewidth of the entangled pair. To do this, they are putting the crystals used to create the entangled photons in an optical cavity, which, when tuned correctly, encourages the production of photons with linewidths many times smaller than that which occur naturally.
They are also exploring various techniques to broaden the linewidth of the light that the memories can accept. The issue is that the same mechanism that broadens the linewidth of the light also creates the main source of noise in their system, washing out the tiny signal of the photon. The team expects to demonstrate the storage of these photons within the next two years.
“The other issue, that of heralding, is a much more difficult feat that still has many scientific questions left to be answered,” Wallace said. “Due to this, we are still very much in the research phase when it comes to the mechanisms of these quantum non-demolition measurements. We care about this because it doesn’t do much good to have distributed entanglement if you don’t know that it’s there.”
Wallace claims that although the challenge seems insurmountable—trying to measure the presence of a photon without measuring it directly or destroying its quantum state—there are several promising techniques to accomplishing it, two of which their lab is at the forefront of research. The first technique uses external light fields that interact with the atoms slightly differently when a photon has been stored versus when there is no signal there, and the other uses atom-cavity interactions that make the system behave slightly differently when there is a photon versus only vacuum.
“Once the physics has been worked out, a tabletop demonstration of the idea could take place; however, taking this to the real world to what we call the quantum internet is another jump,” Wallace said. “First, we have the problem that almost every quantum memory cannot work with the wavelengths that travel long distances in fiber, the telecommunication frequencies that the modern internet runs on.
“To overcome this, we leverage some telecom transitions in the same atoms that we can store our light in to convert the frequency of the photons to that of which can be stored,” he added. “The frequency conversion has been demonstrated, but we still need to show that we can convert and then store light that has traversed over fiber.
“Since most of the experiments in the lab are on air-ride floating tables in temperature and humidity-controlled environments, for a real quantum internet, we need to move to rack-mounted equipment in less-than-ideal data centers,” Wallace said. “We’re developing entanglement sources and quantum memories that are designed to operate in data centers, and we also have an operational detection node in a data center that we have been experimenting with.”
There’s little doubt that there will be a quantum internet in the future based on a network of quantum computers that will create, process and transmit quantum states and entanglement. The research team is making significant strides to that end. Expectations are that it will be at least 10 to 15 years before it is a reality.
Advertisement