Automated vehicles embedded in intelligent and interconnected infrastructures are the future of transportation, but there are huge technological challenges that are still unsolved. Nevertheless, the market share of this industry is expected to continuously increase, provided that autonomous vehicles (AVs) can meet the connectivity, safety, and security requirements of the regulatory agencies responsible for the field.
This will include not only self-driving cars themselves but also autonomous transit systems, such as buses and railways, and the infrastructure necessary to accommodate these vehicles. The combination of vehicles and infrastructure is called intelligent transport systems (ITSes).
In this article, we provide an overview of the current ITS industry and discuss how software-defined radio (SDR) devices can be used in the development and integration of ITS infrastructure, including fail-safe command and control of AVs, which require high-speed and low-latency data links to satisfy the time-critical decision-making involved in the self-driving processes.
We also examine how SDRs are essential to implement robust security schemes and encryption algorithms to provide immunity against malicious attacks and RF interference. SDRs are proving to be valuable tools in ITS development.
The current state of AVs and ITS
ITSes are not only capable of making transportation easier but are essential to increase safety in traffic, improve congestion problems, and help tackle the growing greenhouse gas emissions across the globe. The introduction of wireless communication systems and an intelligent process of information allows for more efficient, safer, and more sustainable transport systems by providing service-based network infrastructures to enable more informed and coordinated decision-making in AVs. These systems compose the ITS industry, which is an emerging market that includes self-driving vehicles, road infrastructure, and RF devices for the wireless network.
There are several different applications of ITS devices, from basic management systems — such as car navigation, traffic signal control, container management systems, variable message signs, automatic number plate recognition, and automatic incident detection — to more advanced applications that integrate live data and feedback from different sources, including parking guidance, weather information, and bridge de-icing monitoring. ITS can also be used to integrate road-enforcement equipment, including speed-limit monitors, red-light cameras, bus-lane cameras, level-crossing cameras, and radars.
Furthermore, ITSes are crucial to enable advanced driver-assistance systems (ADAS), which use wireless sensors, cameras, and artificial intelligence to detect obstacles and driving errors to automatically assist the driver, with several levels of autonomy (see Figure 1). For proper functioning of all these features, functions must support vehicle-to-vehicle (V2V), vehicle-to-infrastructure (V2I), and infrastructure-to-vehicle (broadcasting) communications.
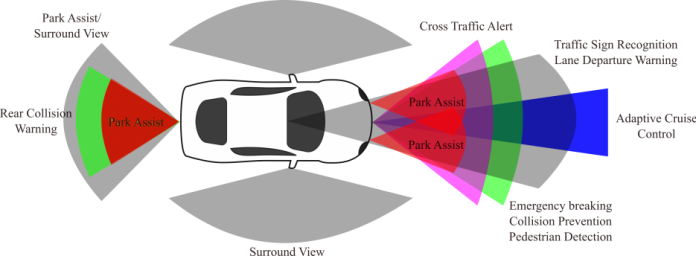
Figure 1: ADAS sensors include cameras (gray), ultrasound (red), LiDAR (green), short-range radar (purple), and long-range radar (blue). (Source: Per Vices)
There are several benefits in integrating ITS in real-world infrastructure. First, logistics operators can use all the data collected through sensors and cameras to plan, schedule, and control the vehicle flow for traffic management and congestion prevention. This intelligent traffic control can also minimize the number of accidents on the road — that correspond to a big portion of deaths and injuries globally — by implementing speed control and distraction-detection measures. Finally, ITS can be used to coordinate vehicles to reduce the greenhouse gas emissions and air pollution caused by traffic by reducing traffic congestion and providing smarter solutions in public transportation.
Despite the potential of solving major transportation issues, there are still several technological limitations that make it difficult to integrate ITS systems in the infrastructure of most cities, and progress in this area has been significantly slow. An alternative approach in telecommunications must be adopted to address the huge amounts of sensor and vehicle data being transmitted continuously, while also ensuring safe, reliable, and secure information exchange.
For instance, the future of ITS may rely on vehicle-to-everything (V2X) 5G networks, where data is exchanged between on-board units (OBUs) in vehicles and roadside units (RSUs). To accelerate this development, the 5G-IANA project in Europe aims to design and implement the Automotive Open Experimental Platform (AOEP), which is a prototype platform for V2X experimentation. Dedicated standalone command and control of AVs, not connected to 5G at all, are also possible and will be discussed in the next sections. Figure 2 shows a diagram for ITS implementation, highlighting the service requirements.
To provide safe and reliable services for ITS applications, mobile communication systems must be able to satisfy several technological requirements. First, many flexible modems are required, each one able to implement several communication protocols and allow for updates on the fly so that the infrastructure can adapt to different user equipment, sensors, and vehicles.
Providing flexible communication while keeping the network safe and reliable is a huge challenge for communication systems, especially because Wi-Fi and LTE technologies are not optimized for mobile applications. Furthermore, the RF devices must comply with a variety of size, weight, and power (SWaP) requirements to address different wireless deployment, from remote stations powered by solar energy to on-board communication units in small vehicles.
Low-latency communication protocols are mandatory, as the decision-making in AVs is crucial for safety and efficiency. This low latency is tightly related to the processing power of the RF units, which must handle huge amounts of simultaneous data that require heavy parallel computation, so FPGA-based signal processing is highly desirable. Finally, the RF devices must provide reliable, safe, and secure communication protocols, which include data encryption, data access protection, fail-safe operations, and redundant functionalities.
Software-defined radio for ITS
SDRs are one of the most important RF developments in recent history, embedding most of the radio functions, which are implemented with analog circuits in conventional systems, in the digital domain. This approach introduces a whole new level of flexibility, robustness, and precision when compared with traditional radio, while enabling functionalities that are possible only in the digital realm.
In its essence, an SDR is a transceiver composed of two main building blocks: a radio front end (RFE) and a digital back end. The RFE provides all the analog functions required for antenna coupling, filtering, and amplification and is designed to work with a wide tuning range. In fact, the highest-bandwidth SDRs in the market implement RFEs with instantaneous bandwidth of 3 GHz over several independent Rx and Tx channels. Each channel contains its own ADC/DAC, enabling multiple-input multiple-output (MIMO) operation.
The digital back end consists of an FPGA with on-board DSP capabilities, including modulation, demodulation, up-/down-converting, data packaging, and networking capabilities. It is also able to be programmed on the fly, which means that an SDR-based RF device can be completely repurposed for an application or adapt to different situations (such as changing the modulation scheme and communication protocol) without any hardware replacement. In a network perspective, these devices can adapt in real time to different PHY and MAC layer mechanisms, allowing the ITS infrastructure to be easily integrated into legacy equipment and allow the implementation of novel units, such as sensors and vehicles.
In the ITS context, it is expected that SDRs will play a major role as adaptable modems, able to adapt to a variety of different modulation schemes. In this scenario, vehicle manufacturers can implement software updates to allow users to comply with the local infrastructure, as well as service providers to incorporate new users and novel technologies without needing to upgrade the hardware.
SDRs can be implemented as intelligent RSUs for both 5G integrated networks and standalone controllers for local public systems. One example is the NXP Semiconductors QorIQ LS2084, which is a system-on-chip that can be incorporated into RSUs to process radar, vision, analysis, and communication for V2X systems.
SDRs are also an integral part of network base stations, providing network backhaul via Ethernet to multiple wireless user equipment, allowing tight integration with existing networks and enabling the implementation of vehicular ad hoc networks (VANETs), which encompass V2V, V2I, vehicle-to-pedestrian (V2P), and vehicle-to-network (V2N).
There are several advantages of implementing SDRs on ITS devices. First, the high level of flexibility and upgradability is highly desirable in AV infrastructure, as SDRs can be completely repurposed on the fly and designed to meet several SWaP specifications, satisfying most network unit and user equipment requirements. This feature also enables the device to work with a variety of different data protocol standards, as well as supports future updates without any hardware replacement.
The FPGA back end also provides a means for parallel computation with extremely low and deterministic latency, which results in near-real–time signal processing and interfacing. The parallel computation of FPGAs is essential to support the heavy processing involved in VANETs.
SDRs also facilitate the collaborative research between multiple groups by implementing open-source development toolkits, such as a GNU Radio, and allowing remote access via Ethernet, which is essential in RF testbeds. Security can also be significantly improved using SDRs, allowing ready-to-use encryption schemes, key generation, error-correction algorithms, and fail-safe transmissions.
Research shows SDR benefits
There is a wide variety of smart and exciting SDR implementations in ITS and AV equipment, both in the industry and academics. We selected two studies that demonstrate very well the benefits of using SDRs in these systems.
Haziza et al.1 proposed a multi-technology cooperative ADAS using SDR-based equipment for connected vehicles. It was able to simulate and evaluate the performance of both V2V and V2I communications under significant road-safety situations. The system was developed as a proof of concept to verify the feasibility of implementing SDRs in a cooperative ADAS to improve and enhance the performance of the network.
Bloessl et al.2 presents another interesting study involving SDR OBUs, where the authors developed an open-source IEEE 802.11p transceiver to prove the feasibility of applying FPGA-based SDRs on VANET systems. They used the frame error rate to validate the implementation of IEEE 802.11p, measuring it over an AWGN channel.
Furthermore, extensive interoperability tests were conducted with both WLAN cards and the SDR-based IEEE 802.11p prototypes, showing that the reconfigurable physical layer provided by the SDR can properly communicate with different commercial WLAN standards, as well as allow easy modification and debug. Experiments demonstrated the capability of working with unsynchronized clocks in a real-world environment, evaluating the performance of both the receiver and transmitter, which further supported the claim of easy integration and interoperability. The design is available under open-source licensing to enable the reproduction of results and incentivize its use in the VANET industry.
Conclusion
Autonomous vehicles and connected cars are the future of transportation, with the potential to solve several challenges in modern society, including congestion, accidents, and greenhouse gas transmission. By gathering enough data from sensors and processing information through smart algorithms and on-board computers, transport operators can make better decisions in real time to improve the efficiency, safety, integration, and security of transportation systems.
However, there is a long road to travel, which entails a handful of developments in the wireless communication field. To support ITS devices and AVs in real time, it is essential that the network is composed of reconfigurable and upgradable modems and RF units based on SDRs, which are the main building blocks of software-defined networks.
Either as part of 5G infrastructures or as standalone controllers, SDRs can offer several benefits to ITS devices, including easy integration and interoperability with both legacy and modern RF systems, flexible and adaptable functionalities, native Ethernet interfacing with networks and host computers, low-latency parallel computing through FPGAs, on-board MIMO capabilities, and compliance with a variety of SWaP requirements.
Furthermore, the inherent digital environment of SDRs allows easy implementation of smart algorithms, encryption systems, complex communication protocols, and integration with host software for control and data processing, such as a GNU Radio. To demonstrate this potential, two studies involving SDRs for V2V and V2I communication platforms were described, both obtaining excellent results in terms of performance and interoperability.
References
1Haziza et al. (May 2013). “Multi-technology vehicular cooperative system based on Software Defined Radio (SDR).” 5th workshop on Communication Technologies for Vehicles, Nets4cars, France, pp. 84–95.
2Bloessl et al. (2017). “Performance Assessment of IEEE 802.11p with an Open Source SDR-Based Prototype.” IEEE Transactions on Mobile Computing, Vol. 17(5), pp. 1162–1175.
Advertisement