NTT Corp. (NTT) and Okayama University have developed a solution to the increasing problem of IoT signal interference by creating a topological ultrasonic circuit. Claimed as the first of its kind, the gigahertz ultrasonic circuit enables stable signal transmission via ultrasonic filters for wireless communications in complex IoT networks. The breakthrough was achieved by developing miniature, energy-efficient and high-performance ultrasonic filters, creating a circuit that propagates gigahertz ultrasonic waves without interference-causing backward reflection, or backscattering.
The solution is based on the principle of topology that makes it possible to freely control the flow of ultrasonic waves in microscopic spaces on semiconductors without signal reflection. Creating a topology-based ultrasonic circuit approach, in addition to reducing backscattering, it was possible to miniaturize the size of an ultrasonic filter to less than 1/100 of the size of a conventional ultrasonic filter. This approach enables the miniaturization, integration and multifunctionality of ultrasonic filters used in wireless communication terminals, according to the research team.
Existing ultrasonic filters have a single filter function per device, while topological ultrasonic filter circuits can integrate multiple filters on a substrate.

Schematic diagram of an existing ultrasonic filter (top) and a topological ultrasonic circuit (bottom) (Source: NTT Corp.)
The collaboration involved combining an ultrasonic phononic crystal, microscopic holes and waveguides so that the gigahertz ultrasonic circuit could effectively address signal interference. Okayama University brings to the table its acoustic topological structure design, while NTT fabricated the ultrasonic circuit.
A phononic crystal is a periodic composite structure made from a periodic array of inclusions and that exhibits absolute bandgaps whereby propagation of elastic waves is prohibited in any direction. Tailoring their band structure enables the control and manipulation of elastic waves, as well as functionalities such as sound isolation, filtering and signal processing.
A microscopic hole in the unit cell is a combination of four circular holes easily reproduced by micromachining techniques. Ultrasonic waves traveling through the ultrasonic circuit are protected by topological order dictated by the shape of these periodic holes, and the ultrasonic waves show stable propagation without reflection, no matter the shape of the waveguide.
NTT’s ultrasonic circuit features a 2D periodic elastic body in which the holes with threefold rotational symmetry are arranged in a semiconductor thin film. By simply rotating counterclockwise or clockwise, it is possible to achieve an elastic structure with a different topology.
The waveguide through which ultrasonic waves travel is made up of edges sandwiched between clockwise and counterclockwise regions with different topologies. The topological structure on both sides has valley pseudospins that rotate in opposite directions. Ultrasonic waves penetrating the edge are pushed out by vortices on both sides. Even with a bend or defect in the waveguide, they do not reflect backward, known as valley pseudospin-dependent conduction.
The creation of the ultrasonic circuit was necessary for several reasons, according to the researchers. Signal filtering in wireless communications traditionally uses a surface acoustic wave (SAW) filter. However, as the IoT expands, to deal with increased backscattering, more filters must be integrated, resulting in a device size problem.
“In contrast to the current SAW filters, where the filtering band property is determined by their electrode geometry, phononic circuits consisting of artificially engineered periodic structures enable acoustic waves to be guided in a sub-micron-scale narrow channel and the filtering frequency to be designed by engineering periodic structures,” said Daiki Hatanaka, senior research scientist at NTT Basic Research Laboratories.
“We can integrate multiple acoustic filters in a single chip of the phononic circuit, saving space on wireless devices,” he added. “Currently, we aim to develop integrated phononic filtering systems using just five to 10 filters.”
When an external ultrasonic wave is applied to the edge of this structure, valley pseudospin is generated, which rotates in opposite directions, and ultrasonic wave propagation proceeds cleanly along the edge in one direction.
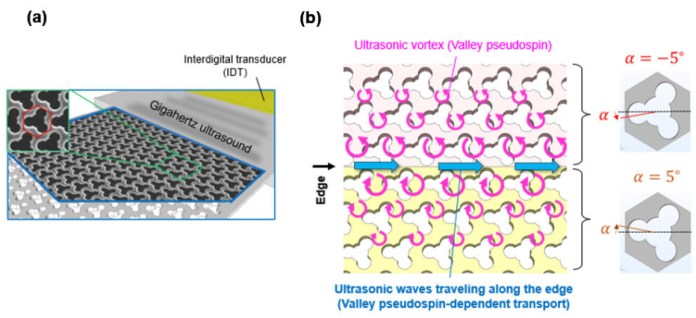
Electron micrographs of a fabricated device (a) and ultrasonic waveguide (b). Backscattering is eliminated at the boundary between two regions with different pseudospin rotation directions (different topologies), and there is a stable propagation of ultrasonic waves. The internal holes are tilted 5° clockwise (yellow) and counterclockwise (pink). (Source: NTT Corp.)
A closer look at valley pseudospin
Waveguide structure involves two types of topological structures with periodic holes tilted 5° counterclockwise or clockwise. The application of an external ultrasonic wave to the edge creates valley pseudospin, rotating in opposite directions, and ultrasonic wave propagation along the edge in one direction. Valley pseudospin represents a quantum number when an electron or ultrasonic wave is in a particular valley or specific region in phase space where energy bands of electrons and ultrasonic waves dip. Valley pseudospin-dependent conduction delivers a robust and stable traveling wave protected by topological order. Even if there is a sharp bend, there is no backward reflection.
“The valley pseudospin-dependent phononic conductions have been observed previously, but only in low-frequency acoustic wave [kilohertz] systems in millimeter-scale or more larger components,” Hatanaka explained. “In our present work, we realized the similar conductions with ultra-high-frequency phonon waves [>0.3 GHz] in a tiny chipscale and used them to demonstrate the principle of filter operation.”
Hatanaka further explained that the importance of such phononic devices will further increase when 6G takes hold. Because 6G communication systems require millimeter-wave signals (30–300 GHz), the advantages of phonons with low radiation loss will become apparent, he added, because electromagnetic waves and the related devices at those frequencies will find it challenging to sustain performance improvements due to increasing crosstalk and losses.
The researchers are also developing phononic devices operating in the millimeter-wave frequency range of about 30–120 GHz, demonstrating the principle of basic signal-filtering operations.
Hatanaka claims that a potential challenge is whether miniaturizing only phonon filters will meet the miniaturization and high-performance requirements of future IoT devices. Other components, including amplifiers and frequency converters, also play a role in suppressing signal interference and in picking up desired signals with a high signal-to-noise ratio.
NTT is developing technology to dynamically control phonon waves and demonstrate active phononic devices such as phonon amplifiers and frequency converters. This will enable the integration of all components in an RF front end, as phonon circuits on a single chip, to achieve sub-millimeter-scale miniaturization.
Advertisement